Biodigestion: Transforming Organic Waste into Energy
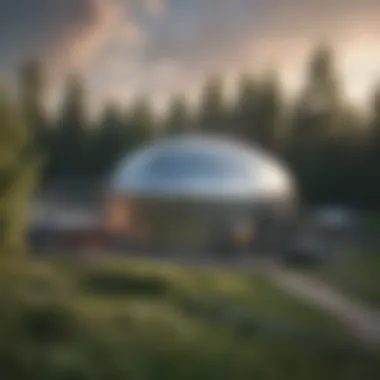
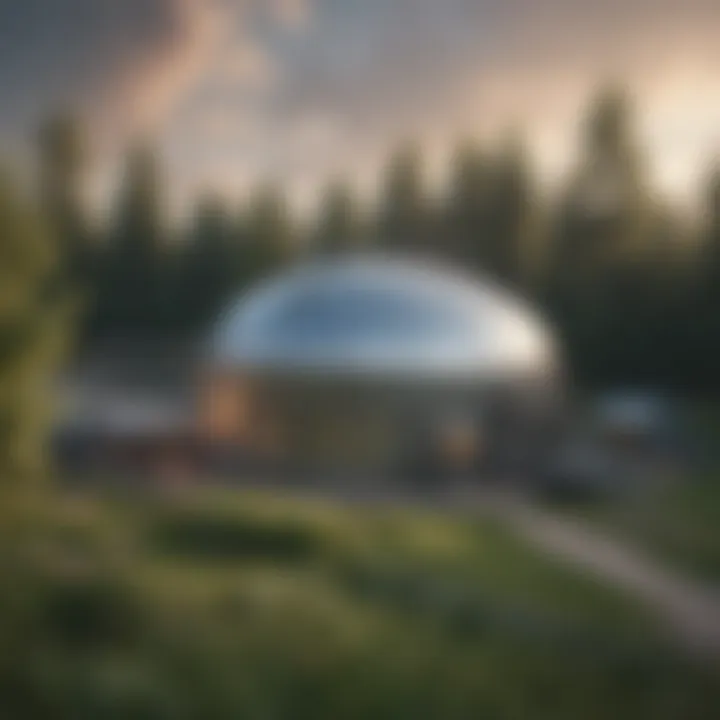
Intro
Biodigestion is involving the transformation of organic waste into biogas and digestate using natural processes. This concept appeals extensively within the context of organic waste management and sustainable agriculture. As we explore this process, it's crucial to grasp its mechanisms, applications, and positive implications for both the environment and energy production.
Understanding biodigestion's operation provides insights into its relevance in contemporary waste management. With increasing urbanization and a rise in food production, the pressure on waste systems intensifies. Therefore, recognizing the significance of organic waste management through biodigestion is vital for promoting more sustainable practices.
Biodigestion emerges as a promising solution, turning potential environmental problems into valuable outcomes.
Overview of the Topic
Biodigestion engages complex microbial processes that transform organic materials into biogas — primarily methane and carbon dioxide — along with digestate, a nutrient-rich substance. Additionally, this technology not only mitigates waste issues but also aids in producing renewable energy, integrating itself into the broader spectrum of sustainability.
From manure to food scraps, biodigestion typically uses a variety of organic materials. This process supports growers, landfill managers, and communities in reducing their carbon footprints. The global discourse on sustainability has propelled interest and research into biodigestion technologies in various regions, particularly focusing on its ability both to reduce greenhouse emissions and generate clean energy.
Current Status and Challenges
The current momentum around renewable energy sources showcases biodigestion as a key player in tackling climate disruptions. However, integrating its application widely faces various challenges:
- Infrastructure needs: Many areas lack the necessary facilities to implement biodigestion systems, hindering progress.
- Public awareness: Misunderstandings about biodigestion can lead to resistance or inaccuracies related to technology and processes.
- Policy support: Effective regulations and policies to foster biodigestion systems are still in a nascent stage in many jurisdictions.
Identifying and overcoming these challenges is fundamental to fully harnessing the significance of biodigestion as a sustainable method for waste management.
Sustainable Solutions
Within the challenge, sustainable approaches significantly reduce waste and optimize natural resource management. Some examples include:
- Sizeable dairy or agricultural farms successfully employ large-scale biodigestion, balancing costs and benefits.
- Many communities in Europe rely on biogas plants as efficient waste reduction mechanisms.
- Additionally, home biodigestion systems provide individuals with creative options for reducing organic waste, showcasing diversity in adaptability to local demands.
Varying successful cases across the globe inform users about achieving successful outcomes with biodigestion technology. The recurring theme remains its economic viability and rapid integration into various market segments.
Impact and Importance
Biodigestion directly influences ecosystems and the practicing communities setting a clear path towards sustainable futures. Proper management strategies not only reduce waste emissions but also enhance ecosystem health, benefiting future generations.
Energy produced contributes to local power grids, relieving some burdens associated with fossil fuels. Promoting conservation will necessitate strengthening biodigestion practices, ensuring communities engage with and invest in renewable energy technologies effectively. The forward march of our societies compels embracing biodigestion practices steadily.
Biodigestion provides a dual approach to waste management and energy production, hence necessary to weave into broader ecological considerations.
Engaging communities is paramount in propelling awareness about biodiversion. Highlighting its multifaceted role in sustainability positively trains focus on practical insights. Moreover, information gleaned catalyzes individual fpr energy-efficient practices resonating throughout communities. Prioritizing exploration in sustainable methods captures the essence of a forward-looking trajectory capable of yielding long-lasting benefits.
Prologue to Biodigestion
Biodigestion is a central process integral to sustainable waste management and energy conversion strategies. It facilitates the transformation of organic waste materials into useful products, such as biogas and digestate. The significance of biodigestion extends beyond waste management, seeping into realms of renewable energy production and ecological conservation. As global initiatives push towards sustainable practices, understanding biodigestion is essential for researchers, policymakers, and conservationists seeking practical solutions to environmental concerns.
Definition and Importance
Biodigestion, commonly termed anaerobic digestion, refers to the biological decomposition of organic matter by microorganisms in the absence of oxygen. The output of this process can be energy-rich biogas, composed mainly of methane, and nutrient-rich digestate that can enhance soil quality. This technology is pivotal for waste, especially organic waste; it mitigates landfill reliance and greenhouse gas emissions.
The importance of biodigestion also lies in its multifunctionality:
- Energy Generation: Producing renewable energy that can be integrated into existing energy systems.
- Nutritional Value: Providing digestate that can be utilized as an organic fertilizer, enhancing the soil.
- Environmental Impact: Reducing the overall carbon footprint by decreasing reliance on traditional energy sources.
Additionally, bios digestion can lock in carbon and maintain soil health, aligning perfectly with goals related to sustainability.
Historical Context
The history of biodigestion traces back thousands of years, with rudimentary forms of anaerobic digestion dating to ancient civilizations that recognized the utility of decomposing organic matter for agricultural richness. Historical records suggest that some of the earliest applications were seen in cultures utilizing manure for fertilization.
Fast forward to the 19th century, as scientists began to explore microbes' roles in decomposition. The formal study of biodigestion gained momentum in the 20th century, particularly during the energy crisis of the 1970s, when interest in alternative energy sources surged. Since then, the technology behind biodigestion has advanced significantly. Today, it embodies an effective merging of biological science, engineering, and sustainable practices as societies worldwide alleviate waste issues and pursue greener energy solutions.
As biodigestion gains recognition, its continued evolution hints at growing public participation and institutional investment. Research surrounding biodigestion systems, legislation, and policy continues diverging as emerging technologies and environmental needs dictate focus. Understanding this historical context forms a foundational perspective from which present and future endeavors must be approached.
The Biological Process
Understanding the biological process of biodigestion is vital for grasping the fundamental principles behind waste management and energy generation from organic materials. This process takes place in an anaerobic environment where bacteria, without oxygen, break down complex organic matter. The insights gained from studying this process fuel advancements in technology and environmental sustainability. Assigning value to waste materials is an essential step toward improving resource recovery and promoting renewable energy production.
Microbial Community Dynamics
Microbial communities are at the heart of the biodigestion process. A multitude of microorganisms occupy the biodigester, working together to convert organic matter into biogas and digestate. These communities comprise various microbes, including bacteria, archaea, and protozoa, each playing distinct roles. Fostering beneficial microbial dynamics is crucial for optimizing the efficiency of digestion operations.
Key aspects include:
- Synergy between species: Specific bacteria collaborate throughout the digestion phases, recognizing that certain groups can enhance the breakdown of certain materials.
- Influence of environmental conditions: Factors such as temperature, pH, and moisture levels impact the health and functionality of microbial communities, which play into their ability to degrade feedstocks.
- Adaptation and stability: Over time, microbial communities can adapt to varying feedstock types, thus ensuring stable gas production.
Contributors must monitor these dynamics closely and understand their fluctuations to enhance system performance.
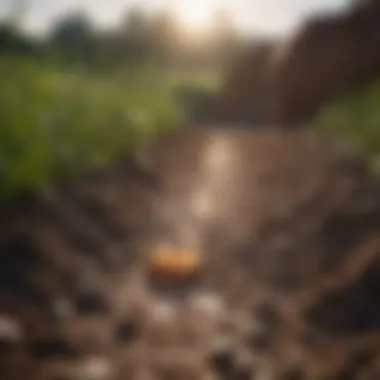
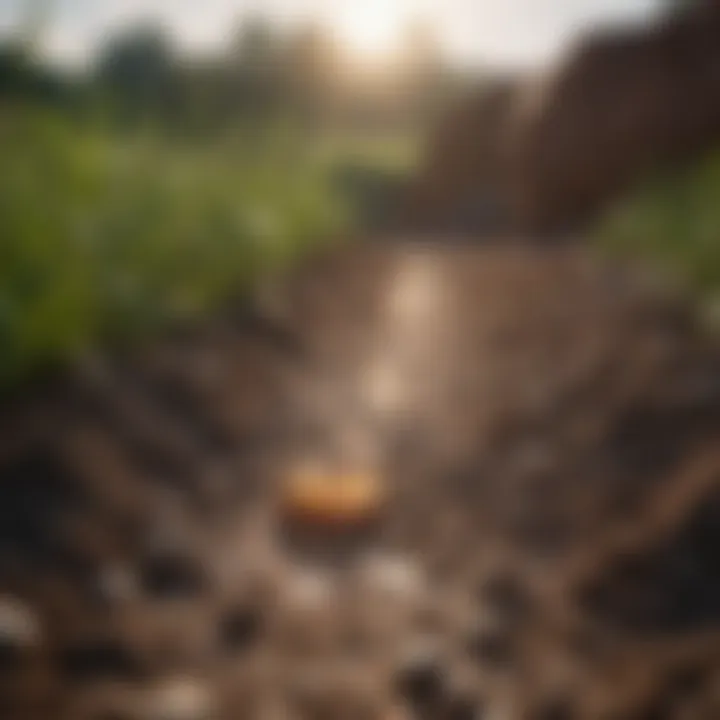
Anaerobic Decomposition
Anaerobic decomposition represents a sequence of systematic phases crucial to biodigestion. There are three primary stages: hydrolysis, acidogenesis, and methanogenesis. Each stage harbors unique processes invoking distinct microorganisms that convert organic materials into usable energy.
- Hydrolysis: Complex molecules such as carbohydrates, lipids, and proteins are broken down into simpler compounds. This stage is typically slow and can be a bottleneck for the overall process.
- Acidogenesis: In this phase, fermentative bacteria convert the hydrolysis products into volatile fatty acids, alcohols, and hydrogen gas. This transition is vital to elevate substrate responses in subsequent phases.
- Methanogenesis: The final stage is characterized by methanogens, specific microbes that convert organic acids and hydrogen into methane and carbon dioxide. The objective here is to maximize biogas output, the most sought after product.
Each of these stages must work in harmony for effective biodigestion. Disruptions in any phase can potentiate reduced biogas output, leading to ineffective waste management.
Biochemical Reactions Involved
Biochemical reactions are pivotal in converting complex organic matter into biogas through various microbial processes. These can be classified into several reaction types that contribute collectively to bioproduct formation.
The principal biochemical reactions include:
- Hydrolytic reactions: Facilitate the breakdown of polysaccharides into simpler sugars which then undergo further fermentation.
- Fermentation reactions: Conclude with the generation of intermediate products such as volatile fatty acids, critical for propelling the subsequent methanogenic step.
- Methanogenic reactions: Encompass the conversion of carbon dioxide and hydrogen into methane. This step is essential for producing biogas as an energy source.
Researchers continue to explore modification in reaction pathways to enhance the yield of desired products. Understanding these unique biochemical processes strengthens the infrastructure of biodigestion systems and contributes to developing more efficient energy solutions.
The success in biodigestion hinges on applying knowledge of the biological process efficiently. Understanding microbial activities, decomposition timelines, and specific biochemical reactions can lead to substantial advancements in organic waste management.
By grasping the intricate workings of these biological facets, practitioners can elevate their strategies and innovate solutions in sustainable practices.
Types of Biodigestion Systems
Understanding the various types of biodigestion systems is essential in optimizing the conversion of organic matter into biogas and nutrient-rich digestate. Each system has its own unique characteristics, applications, and advantages, which can play a pivotal role in efficient waste management and energy production. Choosing the right system largely hinges on factors such as the type of feedstock available, project scale, and local environmental regulations.
Batch Systems
Batch biodigestion systems operate by processing fixed amounts of organic material at specific time intervals. The feedstock is loaded into the digester, and once digestion is completed, the materials are removed before the next batch is introduced.
Benefits of Batch Systems:
- They are relatively simple to set up and manage.
- Their design allows for better process control.
- These systems can be operated in remotely located areas, where continuous systems might not be feasible.
This system is especially effective for managing smaller quantities of waste or for farms that introduce fresh biomass periodically. However, the limitations it imposes, such as cyclic downtime, can hinder efficiency when dealing with large amounts of biomass.
Continuous Systems
Continuous biodigestion systems maintain a steady inflow of feedstock and outflow of effluent over time. As fresh materials are added, digested material is drawn off simultaneously. This flow ensures that bacterial populations are continually active, which can enhance biogas production levels.
Considerations for Continuous Systems:
- Greater efficiency due to uninterrupted biogas production.
- Scalable according to the size of the project, which makes it adaptable for large-scale operations.
- Requires more sophisticated management compared to batch systems, which may lead to higher operational costs.
Continuous systems are preferable for large facilities producing significant amounts of organic waste, as the consistent digestion process maximizes the output of renewable energy.
Plug Flow Reactors
Plug flow reactors represent an advancement in biodigester technology. These systems ensure that the feedstock moves through the digestion chamber in a ram-like manner, which maximizes retention time and contact with microorganisms.
Key Features of Plug Flow Reactors:
- Excellent for high-solid content feedstocks, allowing efficient treatment of organic matter.
- Improved biogas yield due to optimal flow and mixing of materials.
- Smaller footprints compared to traditional digester designs, promoting spatial efficiency in waste management.
In essence, plug flow reactors are invaluable in scenarios where the feedstock varies significantly in composition. They are an ideal fit for agricultural residuse with higher fiber content or other complex organic materials.
Exploring the types of biodigestion systems reveals their complements to various waste management needs. Each system offers distinct advantages, and selecting the right one signifies positive implications for efficiency and sustainability.
Feedstock Selection
Feedstock selection is a crucial aspect of biodigestion, influencing the efficiency and output of the entire process. Choosing the right organic materials not only determines the quality and quantity of biogas produced but also impacts the sustainability of the biodigestion practice. Inadequate or inappropriate feedstocks can lead to operational challenges and suboptimal biogas yields. Careful consideration must be given to the characteristics of feedstocks, including moisture content, nutrient composition, and biodegradability. The effective selection and preparation of materials can elevate biodigestion to a significant renewable energy source.
Organic Waste Materials
Organic waste materials include a broad range of substances originating from food, yard, and kitchen wastes. These materials are often abundant in urban and rural settings and serve as a readily available resource for biodigestion. Among the key benefits of utilizing organic waste is the potential for waste diversion from landfills, leading to a reduction in methane emissions from decomposing organic matter. Additionally, organic waste is typically rich in energy, making it an ideal candidate for biodigestion.
Practical considerations include:
- Moisture Content: Moisture levels in feedstock should typically range from 50% to 85% for optimal microbial activity.
- Contaminants: All feedstocks should be examined for plastics or metals to avoid equipment damage and maintain digestate quality.
- Homogeneity: Mixing different organic materials enhances consistency and efficiency in digestate digestion, allowing the microbial community to thrive.
By selecting appropriate organic waste materials, a biodigestion system can be tailored to maximize biogas generation and minimize operating issues.
Agricultural Residues
Agricultural residues, such as straw, stems, and leaves, represent another important category of feedstock. These materials are often high in carbon content and can balance the nitrogen-rich components of food waste for optimal biogas production. Their uniform availability and lack of treatment processes make agricultural residues an economical option for biodigestion operations. Furthermore, their utilization aids in enhancing soil health through nutrient recycling and reduces burning practices that contribute to air pollution.
Key points concerning agricultural residues include:
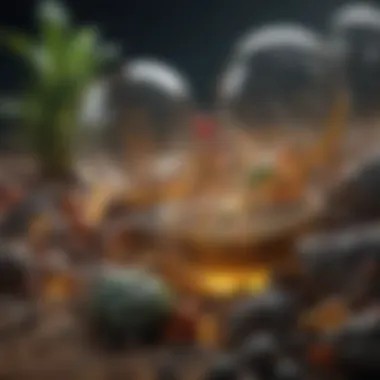
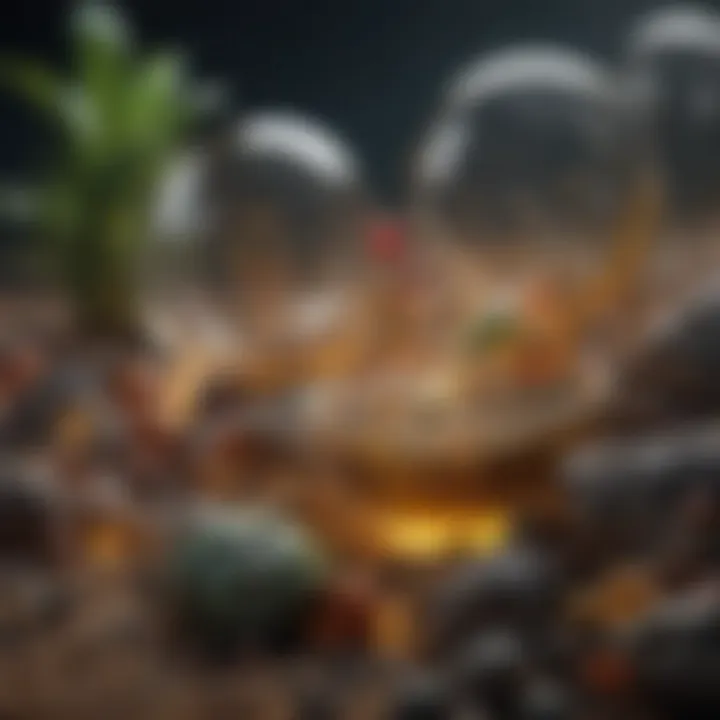
- Nutrient Richness: They provide essential nutrients, promoting healthier microbial communities in biodigesters.
- Seasonality: Their supply can vary with seasonal agricultural cycles, which may necessitate storage facilities or elaborate inventory management.
- Preprocessing Needs: Some residues may need grinding or shredding to optimize multi-phase biodegradation.
Utilizing agricultural residues aligns well with sustainable agricultural practices, contributing significantly to both biogas yields and environmental health.
Food Waste Impacts
Food waste variety is a significant contributor to biodigestion systems, representing an often-discarded resource. In every stage of food production, processing, retail, and consumption, substantial amounts are wasted, leading to both economic losses and environmental harm. In biodigestion, food waste offers a surplus of microorganisms, readily breaking down into valuable biogas and plant-stimulating digestates. Moreover, consumption of food that would typically rot in landfills represents considerable savings in greenhouse gas emissions.
Considerations related to food waste in biodigestion include:
- Composition Variability: The variation in compositions among food waste streams gets a careful evaluation to optimize digestion.
- Contamination Prevention: Protocols must be established to limit oils or chemicals in certain food wastes which may hinder digestion processes.
- Consumer Education: Polling adequate understanding on food repossession from general public can hedge large sources of materials toward diversion for biodigestion.
By integrating food waste into biodigestion systems, a sustainable waste management strategy can be created that yields not just energy, but also environmental benefits.
Biodigestion can greatly contribute to social and commercial initiatives aiming at sustainable waste management while enabling renewable energy generation. Vital future strategies stem from a wise feedstock selection.
Applications of Biodigestion
Biodigestion serves as a multifaceted solution within the domains of renewable energy, agriculture, and environmental preservation. The adaptations and innovations resulting from biodigestion processes assert significant influence over how we manage organic waste and its many byproducts. This section sheds light on the utility of biodigestion in various applications, aiming to showcase its vital role in sustainability and effective waste management practices.
Renewable Energy Production
The quest for clean energy leads to the exploration of biodigestion as a modern method for obtaining renewable energy. Biogas, a product of anaerobic digestion, primarily consists of methane and carbon dioxide, which can be harnessed for various energy needs. In many cases, it can be used to generate electricity or heat.
- Efficiency of Utilization: Biodigestion systems effectively convert biomass into biogas, achieving significant energy yields with relative stability compared to conventional fossil fuels.
- Reduction of Organic Waste: Utilizing organics to produce energy helps alleviate waste issues. By diverting waste materials from landfills, it ultimately reduces methane emissions.
- Scalability and Diversity: From household digesters to large-scale processing plants, biodigestion systems can be implemented on various scales, adjusting to the needs required.
Implementing these energy conversion methods makes a substantial contribution to increasing energy independence and minimizing our carbon footprint.
Soil Fertility Enhancement
Biodigestion is not just about creating energy; it also significantly affects soil health and productivity. The digestate produced from biodigestion often contains many nutrients vital for plants, transforming organic waste into a valuable fertilizer.
- Nutrient Richness: Digestate, the residual bioproduct of the biodigestion process, holds crucial nutrients such as nitrogen, phosphorus, and potassium. These are essential for promoting robust plant growth.
- Soil Structure Improvement: The application of digestate can enhance soil microbial activity and organic matter. This process supports better soil structure and moisture retention.
- Horticultural Usage: Many commercial farms and garden industry professionals often favor digestate as a natural alternative to chemical fertilizers, thereby sustainably enhancing soil productivity.
Efforts are made globally to harness this byproduct, demonstrating the symbiosis between waste management and agricultural success.
Wastewater Treatment Solutions
In the field of wastewater treatment, biodigestion processes are proven effective. By integrating biodigestion into treatment facilities, municipalities can utilize waste in an innovative manner.
- Reduction of Harmful Substances: Biodigestion aids in the breakdown of organic pollutants present in wastewater, rendering the water cleaner and more manageable.
- Resource Recovery: The sludge that remains after the digestion process can also yield additional nutrients and biogas for electricity generation, making it a resource rather than a waste.
- Economic Advantages: Using biodigestion in wastewater treatment can lead to cost savings related to energy bills and waste disposal methods, positively influencing municipal budgets.
Streamlining treatment processes enhances system resiliency while improving public health and environmental quality.
In summary, applications of biodigestion offer diverse benefits that connect energy, agriculture, and environmental conservation, showcasing its essential role within sustainable practices.
Technological Innovations
Technological innovations in biodigestion play a vital role in improving the efficiency and effectiveness of organic waste processing. This ensures not only greater energy production but also enhanced sustainability. Advances in digestion technology allow for the optimization of multiple factors, ranging from microbial community function to reactor design. The real benefit lies in being able to convert a larger quantity of feedstock into valuable byproducts such as biogas and digestate.
Advancements in Digestion Technology
Recent advancements in digestion technology focus on enhancing the anaerobic digestion process. New systems monitor and control temperature, pH levels, and pressure more accurately. Some technologies utilize sensors and data analytics. This attentiveness improves the microbial activity and maximizes biogas yields. Additionally, advanced pre-treatment processes help break down tough organic materials, allowing for a more efficient degradation. Some current technologies include:
- Encoder-driven systems: Help in maintaining optimal operating conditions.
- Microbial fuel cells: Convert organic matter directly into electrical energy.
- Enzymatic hydrolysis: Breaks down complex biomolecules into simpler sugars, boosting methanogenesis.
These technological advancements not only improve the outputs but also reduce operational costs and environmental impacts.
Integration with Other Renewable Technologies
The integration of biodigestion with other renewable technologies can amplify its effectiveness. For example, coupling biodigesters with solar systems allows for partial energy independence in operations. When both technologies are in tandem, solar energy can power digesters and enhance overall energy production.
Additionally, utilizing biodigesters in conjunction with microbial fuel cells can create a dynamic symbiosis. In this setup, waste byproducts from biodigestion can be transformed into additional electricity, further maximizing resource use. This reinforces the concept of a circular economy, where waste materials turn into assets.
"The future of energy lies in integration. Technologies must support each other to drive sustainability further than any could achieve alone."
Overall, technological innovations in biodigestion do not merely lead to enhanced conversion rates of organic material. They pave the way for a unified, more sustainable energy ecosystem that continuously learns and evolves. These advancements are pivotal not only for resource optimization but also for influencing policy and societal acceptance of biodigestion practices. With ongoing research and development, the scope and efficiency of biodigestion systems will continue to expand, making them an essential component of our renewable energy landscape.
Environmental Impacts
Understanding the environmental impacts of biodigestion is crucial, as this process holds significant roles in combating ecological challenges. The process converts waste into usable energy while minimizing harmful emissions. This synergistic approach invokes immediate considerations about its viability and broader implications for sustainability. The focus on managing organic waste can lead to measurable advancements, especially for conservationists and environmentalists.
Reduction in Greenhouse Gas Emissions
Biodigestion plays a prominent role in the reduction of greenhouse gas emissions. Methane, a potent greenhouse gas, is often released during the decomposition of organic matter in landfills. However, biodigestion captures this methane and transforms it into usable biogas. According to studies, replacing traditional waste disposal with biodigestion can result in a significant decrease of greenhouse gases on a national scale.
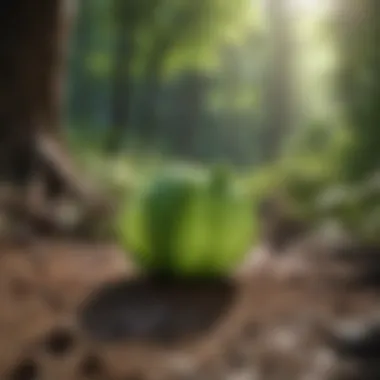
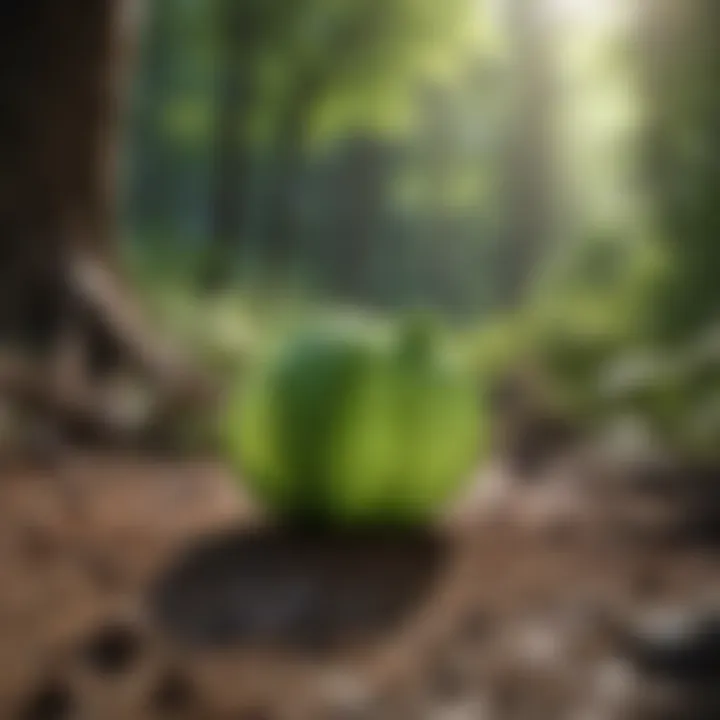
When waste is processed through biodigesters, approximately 60% of methane production is avoided. This change reduces carbon footprints not only for facilities employing biodigestion but also extends to downstream users, lightening the load on our atmosphere. Moreover, anything that organic waste contributes, such as carbon dioxide and other harmful components, can also enter as a reduced factor when properly managed by biodigestion.
Key Benefits:
- Reduction in landfill reliance.
- Decrease in onsite methane emissions.
- Contribution to energy needs, further lowering fossil fuel dependence.
Biodigestion highlights the critical opportunity to tackle climate change by utilizing biogas instead of allowing methane to escape into the environment.
Contribution to Circular Economy
A fundamental principal of sustainability, the circular economy aims to keep resources in use for as long as possible. Biodigestion supports this model effectively by converting waste into energy and nutrients for agricultural use, thus addressing two main issues: waste management and nutrient deficiencies in soil. This not only prompts a reduction in waste but reclaims valuable resource.
When exploring feedstocks for biodigestion, organic waste from agriculture, food industries, or municipal sources becomes critical. The post-digestion material—digestate—serves as a rich fertilizer suitable for soil amendment. The flow of nutrients back into the earth is a significant advancement toward sustainable agriculture.
Contributions:
- Enhances soil fertility, making land more productive.
- Decreases the need for synthetic fertilizers in agriculture.
- Provides an alternative waste reservoir, pressing need under current climate impact assessments,
Challenges and Limitations
Understanding the challenges and limitations of biodigestion is vital for securing its place as a sustainable practice in organics management and energy production. While biodigestion offers significant advantages, it is not devoid of obstacles that can hinder its implementation and effectiveness.
Technical and Operational Hurdles
Technical challenges during the execution of biodigestion systems can affect their performance. These hurdles may include:
- Variability of Feedstock: Different organic materials may yield varied results in biogas production. Feedstocks may be inconsistent both in quality and in composition, thus affecting microbial effectiveness.
- Temperature Control: Maintaining the optimal temperature for microbial activity is essential. Fluctuations can lead to suboptimal digestion and decreased yield of biogas.
- Equipment Failure: Mechanical breakdowns in biodigesters can halt operations. Regular maintenance is essential, yet costs may be prohibitive for some users.
As these technical and operational challenges persist, they require expertise, careful monitoring, and infrastructure that may not be universally available.
Keeping a biodigestion system operational demands precision in engineering and biology, together with continual attention.
Regulatory and Economic Barriers
Regulatory limitations can pose serious constraints on the viability of biodigestion projects. The landscape surrounding environmental regulations is varied and complex, making it difficult for biodigesters to operate.
Economic barriers also exert a pressure on biodigestion adoption:
- Funding Requirements: Initial setup costs for biodigestion are often high. Many stakeholders struggle to acquire funding or incentives to support their projects.
- Market Dynamics and Profitability: The very profitability of products generated can vary. For instance, the by-products of this process may need specific markets for sale, which are not always assured.
These regulatory and financial constraints serve to underscore the multifaceted nature of obstacles to biodigestion. Efforts must be coordinated at multiple levels to enable better opportunities in this significant area of sustainability legislation and initiatives.
Future Prospects of Biodigestion
Biodigestion is at a significant juncture where its potential to address pressing environmental and energy issues is increasingly recognized. The focus on future possibilities reveals how biodigestion can become integral to waste management and sustainable energy production. With input from various sectors, the development of biodigestion technologies becomes a shared objective with direct benefits across myriad domains.
Emerging Trends
Understanding emerging trends is vital for grasping the evolution of biodigestion. Current advances shape the way we approach energy and waste processing, which involves three major aspects:
- Integration of AI: Artificial Intelligence can optimize the biodigestion process in real-time. Predictive analytics can refine feedstock management and enhance the efficiency of gas production.
- Decentralized Systems: Smaller, localized biodigestion facilities are attracting attention. This trend supports community initiatives and reduces energy loss in transmission.
- Waste-as-Resource Concept: The transformation of waste into energy is gaining traction. This global movement underscores the value materials can provide, highlighting the sustainable nature of biodigestion practices.
These trends not only indicate technological evolution, but also engage public interest and policy advancements.
Role in Global Sustainability Goals
Biodigestion aligns intrinsically with global sustainability goals. It specifically plays several roles in this context:
- Emissions Reduction: By producing biogas instead of allowing organic waste to decompose naturally, we mitigate methane emissions, a significant greenhouse gas.
- Resource Recovery: Digestate, a byproduct of biodigestion, can rejuvenate soil health, thus contributing to sustainable agriculture. By doing so, it echoes the principles of circular economy.
- Energy Security: As the demand for renewable energy grows, biodigestion provides a solution. It generates consistent and renewable biogas that contributes to energy diversification.
In summary, through full integration of biodigestion systems, we can make more than just energy – we form a substantial part of environmental stewardship, ensuring ecological balance and enhancing human well-being.
Biodigestion techniques embody a vital component in realizing our ambitions for sustainability. Collective efforts in this field can foster broader acceptance and widespread implementation of eco-friendly practices.
Culmination
The topic of biodigestion converges essential elements in the spheres of organic waste management, renewable energy, and environmental sustainability. Within this comprehensive framework, the conclusion emphasizes the significance of biodigestion as a viable solution to complex ecological challenges. This summary brings together various threads presented in the article and highlights the multifaceted advantages that biodigestion offers.
Summation of Key Insights
Biodigestion transforms organic materials into valuable resources like biogas and digestate. This process not only reduces waste but also generates renewable energy, thus contributing to a lower carbon footprint. The main insights include:
- Organic Waste Management: Biodigestion effectively manages agricultural, food, and other organic waste.
- Renewable Energy Production: It serves as a significant source of biogas, promoting energy independence.
- Soil Fertility Enhancement: The digestate produced improves soil quality by adding vital nutrients.
- Environmentally Friendly Practices: By reducing methane emissions and supporting circular economy principles, biodigestion aligns with global sustainability initiatives.
This combination of benefits underpins the necessity for broader implementation of biodigestion systems in various sectors, including agricultural, municipal, and industrial applications.
Call to Action for Sustainable Practices
It is necessary for businesses, communities, and governments to actively engage in practices that promote biodigestion. Those interested in sustainability should:
- Invest in Biodigestion Technologies: Support the development and installation of biodigestion systems tailored to local needs.
- Educate Others: Spread awareness about the importance of waste reduction and energy sustainability.
- Form Partnerships: Collaborate with other organizations to establish biodigestion initiatives that serve wider communities.
Furthermore, policies should reflect the strategic importance of biodigestion, encouraging systems that facilitate organic waste processing. By collectively fostering a culture surrounding this sustainable practice, stakeholders can significantly impact ecological health and sustainability standards.